Introduction
Guinea fowl are birds derived from the African Guinea coast and are one of the economically important bird originating from the southern part of the Sahara and majorly in West Africa and it is widely domesticated in the region (Crawford, 1990). It is domesticated from wild helmeted guinea fowl (Numida meleagris) and accounts for 3% of global poultry breeds (FAO, 2007). Apart from being a valued source of meat, eggs and feathers, domesticated guinea fowl has value for pest control and alerting intruders (Ayeni, 1983; Moreki and Seabo, 2012). Despite the economic importance of guinea fowl, the extent of its genetic diversity remains an enigma (Adeola et al., 2015). In Nigeria, Guinea fowl is one of the indigenous poultry and also an integral part of rural poultry. A recent report indicated that it is the second most widely domesticated poultry after chicken (NBS, 2012) and found mainly in northern Nigeria. In spite of the abundance and popularity of guinea fowl in northern Nigeria, its sizeable contribution to animal protein production and absence of known taboos and superstitions against its rearing, consumption and marketing; this bird has not received attention it deserves by researchers most especially in regions where it contributes to internal animal production and consumption. The neglect suffered is exemplified in sparse literature on this bird; and studies on its genetic attributes are limited.
Efficient utilization, improvement and conservation of a species or breed are practically impossible in the absence of certain relevant background information of its unique attributes. Characterization of genetic resources of farm animals encompasses all activities associated with the identification, quantitative and qualitative description, and documentation of breed populations as well as its natural habitats and production systems to which they are or are not adapted (Gizaw et al., 2011). Delgado et al., (2001) also identified characterization of a livestock breed as the first approach to sustainable use of animal genetic resources, thus contribute to the importance of characterization of farm animals, while Halima (2007) posited that genetic characterization of the domestic animals is an integral component of the Food and Agriculture Organization’s (FAO) global strategy mainly for the management of farm animal genetic resources and Gholizadeh et al. (2008) stated that genetic characterization of populations or breeds allows the evaluation of genetic variability which is a fundamental element in planning breeding strategies and genetic conservation plans as asserted by Oguntunji et al. (2016).
Considering the fact that approximately 60% of bird species are difficult to differentiate sexually due to their monomorphic nature, even experienced ornithologists have difficulties with sex identification especially in the young and sometimes the matured birds rely basically on morphological analysis of established phenotype (Wu et al., 2007); vent sexing, laparoscopy, steroid sexing or karyotyping (Cerit et al., 2007) or by comparing blood plasma protein profiles between male and female spp. (Suratno et al., 1998). This has been a major challenge to animal breeder interested in captive avian breeding and evolutionary studies. However, sexually dimorphic birds, such as the house sparrow, mallard and collared flycatcher are quite easy to distinguish (Dubiec and Zagalska-Neubauer, 2006).
Like every other avian, Sexing is a major problem militating against efficient selection and breeding in guinea keets and bearing the need to sex at early age in large scale poultry production, to prevent unduly wasting of expensive layer ration on the males. Early in 1975, Awotwi showed that young female guinea fowls tended to have wider pelvic inlet than males, and this difference was evident in birds as early as 2 weeks of age (Awotwi, 1975). In a similar work, Teye et al., 2000 also demonstrated that the presence of a rudimentary phallus in males’ cloaca could be used to distinguish them from their female counterparts. However, these were not confirmed with any molecular or genetic tool coupled with the fact that, this method can be a very slow, particularly when large numbers of birds are involved. Therefore, there is a need to confirm these results and develop other techniques which will facilitate quick and efficient sex determination in guinea keets.
There is inadequate information on the genetic background and diversity of guinea fowl in Kwara state, Nigeria. Lack of regulation on the indiscriminate killing and sales of guinea fowl for meat and other purposes has pose a major threat to the conservative efforts of these important birds. This threat may lead to extinction of some important genetic resources which would be needed for the successful production of guinea fowl. Characterization and conservation of these genetic resources is thus necessary to ensure future food security and wildlife conservation. There is a need to establish a basis for breeding of the almost extinct species with careful selection along a distinct line according to sex. Thus, this study explores the molecular tools to determine a more accurate sexing technique and taxonomic classification of Nigerian domestic guineafowl.
Materials and Method
Source of Experimental Birds
Twenty Guinea fowl keets were purchased from live poultry market in Ilorin, Kwara state Nigeria and taken to a private farm (Fair and Firm Farm Limited, Tanke Oke Odo, Ilorin Kwara state, Nigeria) where they were allowed to acclimatize for two weeks before blood collection. The Experiment was conducted under the ethical approval of the University of Ilorin, Ilorin, Kwara State, Nigeria.
Blood collection and DNA Extraction
Blood sample were collected from 10 randomly selected young Guinea fowl keets. About 2ml of fresh blood was collected by superficial venipuncture of a wing vein of the Guinea fowl using a 2ml syringe needle into the EDTA bottles to prevent coagulation of blood samples. Genomic DNA was extracted from the blood sample using Norgen Kit after according to the Manufacturer’s instruction.
DNA electrophoresis
Extracted DNA was visualized on 1% Agarose gel with Acridine-based chromogen SafeviewTM DNA stain (ABM) in 100 ml of 1 × Tris/Acetic Acid/EDTA (TAE) buffer solution.
Amplification of Exons
Two microliter (2l) of purified DNA template (50–100 ng) was combined with 12.5l Norgen’s 2X PCR master mix (Taq DNA polymerase, reaction buffer, dNTP, MgCl2, KCl and PCR stabilizer), 1ml each of Primer sets (10 pmol of 2550F (5’-GTTACTGATTCGTCTACGAGA-3’) and 2718R (ATTGAAATGATCCAGTGCTTG-3’) in a 20 μL reactions in (BioRad Mycycler Thermocycler). (Griffiths et al., 1998) using an initial denaturation of 94℃, 30 cycles at 94℃ for 30 s, 72℃ for 1 min, and 72℃ for 5 min. Products were visualized in 2% agarose gels, gel isolated (QIAquick Gel Extraction Kit, QIAGEN). Amplified DNA was prepared for sequencing using the “DTCS” method (). Cleaned fragments were sequenced from 5’ ends using the di-deoxy chain terminator method with V3.1 Bigdye terminator chemistry. The resulting sequencing reactions were analyzed on 3700 or 3730 ABI sequencing machines.
Analysis of sequence data
Taxonomic characterization and molecular sexing were accessed at the publicly accessible databaseat and , while the phylogenetic trees were drawn using Clustal W@ https://www.ebi.ac.uk/Tools/msa/clustalo/.
RESULTS AND DISCUSSION
To achieve accurate sexing in avian, previous reports have leverage on the chromosomal sex determination system, however, it has been established that chromosomal system of bird is different from that of mammals. Previous work has identified W-linked sex gen and the gene was found to be encoded by the chromo-helicase-DNA binding gene, where the presence of the W chromosome of most birds was reported (Ellegren, 1996). The Chromo domain helicase DNA (CHD) proteins have the ability to act as overall transcription regulators at the chromatin level, a function suggested by their unusual property of containing three conserved domains, each member of a discrete gene family, implicated in DNA-protein interactions. Like the human, at least four CHD genes are found in the avian W-linked gene is closely related to the one designated CHDI (Woodage et al., 1997); thus, they propose that CHD1W should be used as gene symbol for the avian gene. The situation is however complicated by the fact that CHD1W exists in a second but not W-linked copy, apparently also present in most bird. In birds, females are heterogametic (ZW) while males are homogametic (ZZ), and sexing can thus be made by the detection of the W chromosome or W chromosome sequences in a sample of unknown sex. Since their description these markers have been utilized for sex determination in several species of monomorphic birds (Clinton et al., 2001; Cerit and Avanus, 2007; Harvey et al., 2006 and Kalina et al., 2012). Thanou et al., (2013) applied CHD based markers for accurate sex determination of three of European Phalacrocoracidae species. Liu et al., (2010) applied CHD based primers for accurate sex determination of chicks and embyos in Chinese game cocks. Sulandari and Zein (2012) demonstrated that CHD based primers can be utilized to determine sex of 56 Indonesian wild birds. Vucicevic et al., (2013) successfully applied PCR based on CHD specific primers for sex determination of 50 species of birds after evaluating the method on 58 species. The existence of two avian CHD I genes is both problematic and advantageous from the perspective of molecular sexing. When analysed with PCR as done in this study and also the current method of choice in these types of studies, the similarity of the two CHDI genes implies that it is difficult to amplify only one of the copies. Obviously, some protocol for distinguishing sex is needed in order for a female bird to be clearly identified and the problem of false negatives is avoided thus making selection at younger age easier.
Band Pattern Sexing
High molecular weight DNA bands were observed on 0.8% agarose gel (Figure 1) indicating genomic DNA from guineafowl blood sample. Amplification of both Z and W alleles were also observed in all birds, the female bird shows two (2) bands of about 150 bp apart (A) while the male birds only shows a single band of about 500 bp indicated by (B).
Amplification of both Z and W alleles in all birds using standard PCR and the purity of the template DNA was observed to play significant role in the integrity of the PCR product. Low yield in terms of amplicon concentration and intensity on agarose was observed as well as poor sequence spectra. Some cases of uncertainty such as birds that were considered female base on the absence of helmet at the young age as conventionally assumed were clarified showing single band on agarose and were further subjected to sequence analysis where it was revealed to have more than 88% identity with chromo-helicase DNA binding protein gene I (CHD1) when compared with more than fifty (50) avian species. The polymerase chain reaction (PCR) results from male and female guinea fowl blood samples also shows very different results on sequence because a single DNA band was observed for males, while two were seen in females.
Quantification of DNA Isolate from the helmeted Guinea fowl
Spectrophotometric analysis of the isolated blood genomic DNA revealed the total concentration of DNA obtained per sample was about 234 ng/l with appreciable % purity, indicating samples were pure for polymerase chain reaction (PCR).
Amplification of Gene
The primer set for the 12s rRNA gene were used for taxonomic sequence analysis while a single band of about 1,800 bp (2550F/2781R) which is the band for male and double bands which were about 150 bp apart for the female guineafowl were sequenced using CHD.
Taxonomic characterization
Nucleotide-Nucleotide (blastn) blast obtained for the 12s rRNA sequence revealed between 1,789-2,216 bp, returning with 137 hits of organisms belonging to the Galliforoems, twelve (12) of which were in the class Numididae among which were N. meleagris (8); A. vulturium (3); and G. pucherani with scores 388, 337 and 326 respectively. Meleagris ocellata and gallopavo had 333. The sequence had 98.20% identity with four (4) Numidia spp. N. meleagris partial 12s rNA gene (AM902518.1); N. meleagris mitochondrial partial 12s rRNA gene (AJ490506.1); N. meleagris 12s mitochondrial ribosomal RNA, small subunit, mitochondrial gene partial sequence (U88016.1). Multiple sequence analysis and subsequent phylogenetic deduction reveal the sequences were closest to Numidia meleagris 12s mitochondrial ribosomal RNA, small subunit, mitochondrial gene partial sequence (U88016.1). The phylogenetic tree (Figure 3) shows that Guineafowl keets sequenced is most related to the Numida meleagris 12S mitochondrial ribosomal RNA, small subunit, mitochondrial gene, partial sequence with Accession number U88016.1.
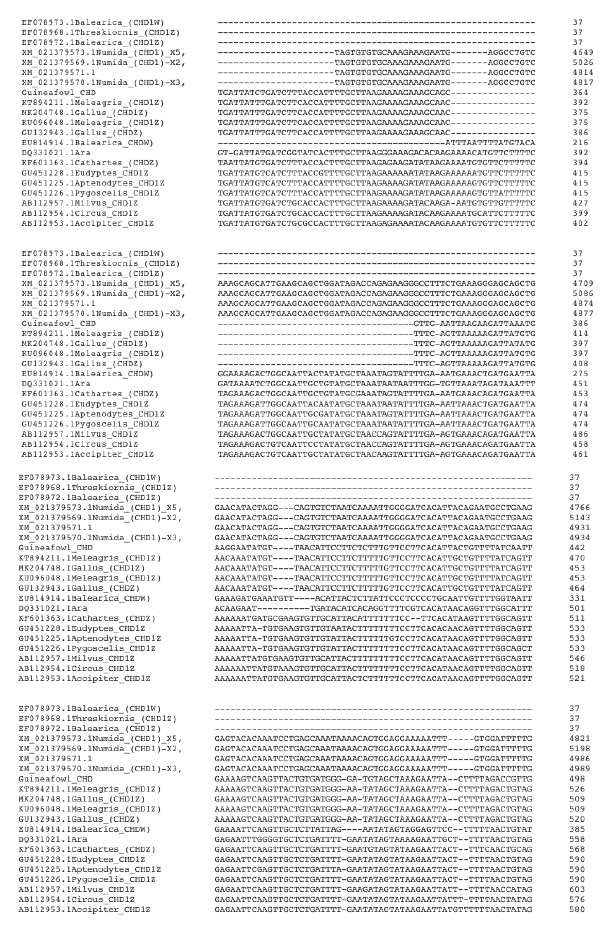
Figure 2a. Multiple Sequence Alignment of guineafowl chromo-helicase-DNA binding protein (CHD) gene sequence along with 20 most related sequences using clustalw on the European Bioinformatics Institution web site https://www.ebi.ac.uk/Tools/msa/clustalo/. The alignment shows Nucleotide substitution/ insertion/deletion. Asterisk is used to indicate complete alignment between query and aligned sequence.
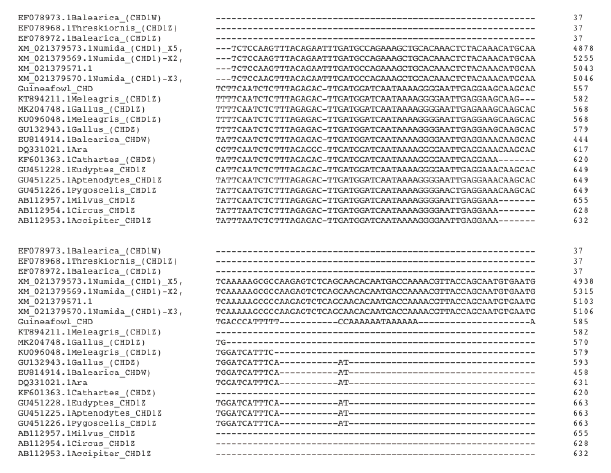
Figure 2b. Multiple Sequence Alignment of guineafowl chromo-helicase-DNA binding protein (CHD) gene sequence along with 20 most related sequences using clustalw on the European Bioinformatic Institution web site https://www.ebi.ac.uk/Tools/msa/clustalo/. The alignment shows Nucleotide substitution/ insertion/deletion. Asterisk is used to indicate complete alignment between query and aligned sequence.
Multiple Sequence Alignment of guineafowl using chromo-helicase-DNA binding protein (CHD) gene sequence along with 20 most related sequences is as shown in Figure 4Figure 4a: Multiple Sequence Alignment of guineafowl 12s rRNA sequence along with 16 most related sequences using clustalw on the European Bioinformatic Institution web site . The alignment shows Nucleotide substitution/insertion/deletion. Asterisk is used to indicate complete alignment between query and aligned sequence.
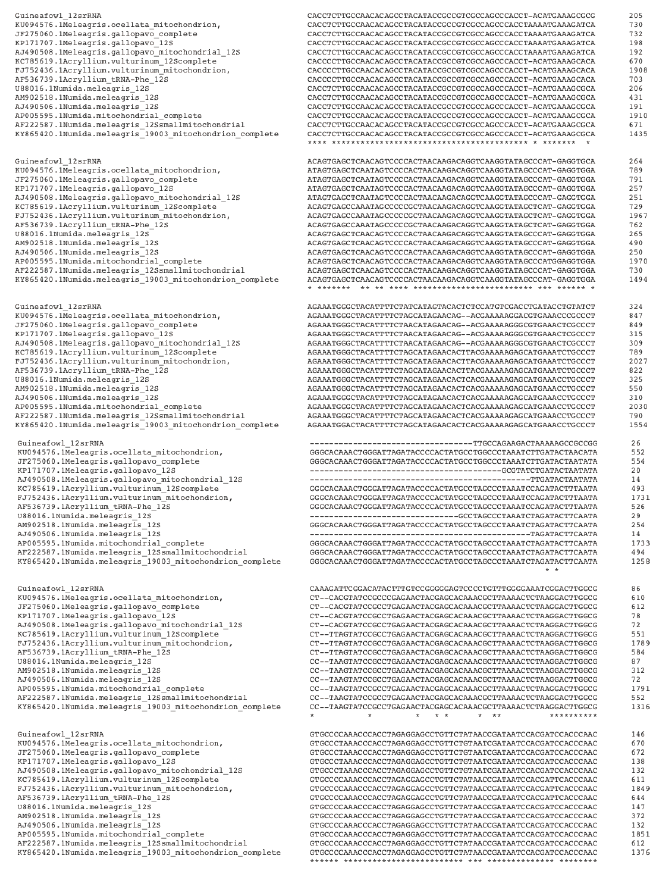
Figure 4a. Multiple Sequence Alignment of guineafowl 12s rRNA sequence along with 16 most related sequences using clustalw on the European Bioinformatic Institution web site https://www.ebi.ac.uk/Tools/ msa/clustalo/. The alignment shows Nucleotide substitution/insertion/deletion. Asterisk is used to indicate complete alignment between query and aligned sequence.
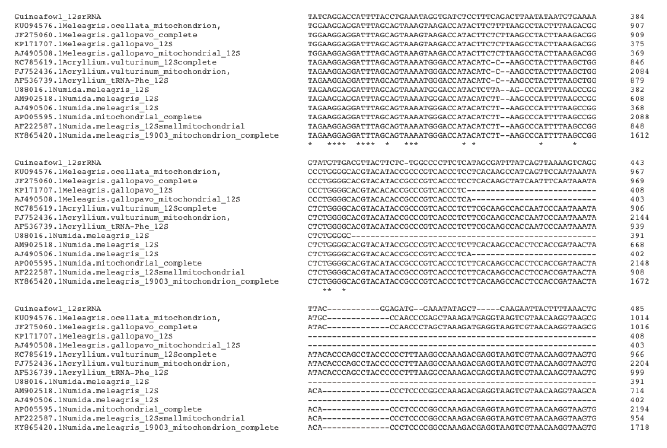
Figure 4b. Multiple Sequence Alignment of guineafowl 12s rRNA sequence along with 16 most related sequences using clustalw on the European Bioinformatic Institution web site https://www.ebi.ac.uk/Tools/ msa/clustalo/. The alignment shows Nucleotide substitution/insertion/deletion. Asterisk is used to indicate complete alignment between query and aligned sequence.
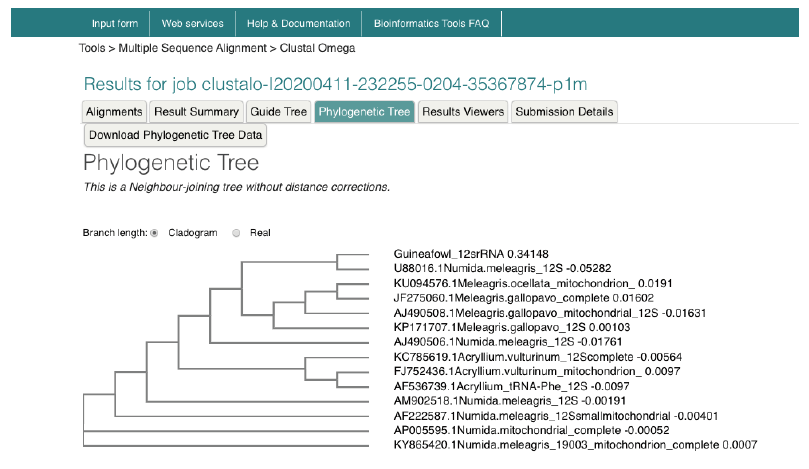
Figure 5. Phylogenetic tree of guineafowl 12s rRNA sequence along with 16 most related sequences using a Neighbour-joining tree without distance corrections on the European Bioinformatic Institution web site https://www.ebi.ac.uk/Tools/msa/clustalo/. The phylogenetic tree shows Guineafowl is most related to the Numida meleagris 12S mitochondrial ribosomal RNA, small subunit, mitochondrial gene, partial sequence Accession no. U88016.1.